About the Auriga simulations
We outline the motivation for the simulations in relation to some key scientific areas of galaxy formation, and describe the details of the simulations including the galaxy formation model.
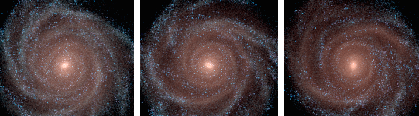
Summary for the non-expert
The history of the Milky Way is written in the starlight. Galaxies like the Milky Way contain more than 100 billion stars. Their positions, motions, and chemical compositions tell us a story about how the Universe and the galaxies and stars within it formed and evolved since the beginning of time. Nowadays, we are observing a lot of stars in our Galaxy, which contain a lot of information the same way fossils in the ground record how life has evolved here on Earth. But the stars we see now tell a much more nuanced and sophisticated story that cannot be disentangled without computer simulations to tell us how a wide range of physical processes operated on unfathomable scales since the Big Bang almost 14 billion years ago.The Auriga project embraces this challenge head-on. The simulations begin just after the Big Bang, and include many physics, such as gravity, dark matter, black holes, and supernovae. We end up with a sequence of snapshots that we can scrutinise in order to see how stars form and galaxies grow at different epochs, and pinpoint the phenomena that drive the structures that we see today.
Motivation
A major goal of theoretical astrophysics is to understand how the observed Universe came to be, and in so doing, to understand the important physical processes of galaxy formation. The standard model of cosmology, Lambda Cold Dark Matter, is well-constrained by early Universe observations such as the CMB, and provides remarkably precise initial conditions for the problem. The difficulty lies in following the non-linear evolution of structure formation over the history of the Universe well enough to assess the drivers of galaxy formation and make observational predictions. Now, in the age of high performance computing, numerical simulations are proving to be a powerful technique to accomplish this formidable task. Both dark matter only (gravity without baryons) and full hydrodynamic simulations have achieved impressive results, predicting the hierarchical growth of structure, and, perhaps most crucially, the importance of stellar and black hole feedback in curtailing star formation. Furthermore, the resulting galaxies reproduce a number of key observables, such as the stellar mass function and realistic galaxy populations: the Hubble sequence from irregulars to giant ellipticals.
An important step towards our understanding of galaxy formation is to simulate successfully the formation and evolution of Milky Way mass disc galaxies in a fully cosmological context. From a cosmological perspective, Milky Way mass disc galaxies are of particular interest because they lie between the low and high mass regimes thought to be dominated by supernova and active galactic nuclei (AGN) feedback, respectively, and are therefore ideal targets to test the models of feedback designed to reproduce whole galaxy populations. Moreover, the simulations allow detailed analysis of the properties and coupled evolution of galactic baryonic material and dark matter of Milky Way-mass galaxies, including their satellites and surroundings. This can be used in conjunction with a wealth of observations such as SDSS, BlueDisk, CANDELS etc. to make statistically relevant observational predictions about a range of physical phenomena important in the formation of galaxies.
In the context of the Milky Way itself, fields such as Galactic archeology, that aim to determine the formation history of the Milky Way from chemo-dynamical information of many individual stars, are growing rapidly thanks to the multitide of data provided by surveys such as RAVE, APOGEE and Gaia. High resolution simulations that are sufficiently accurate to probe a wide variety of physical process of both internal and external origin can then constrain, and be constrained by, scenarios of formation history of the Milky Way, and are therefore very useful in this endeavour.
Simulation details
Simulation sample and resolution
Only in recent years have cosmological 'zoom' simulations been able to reproduce observational properties of Milky Way mass galaxies, with early attempts resulting in overly massive stellar bulges, sub-dominant disc components and highly peaked rotation curves. However, there exists significant disparity in the results obtained from the same initial conditions and parameters with different simulation codes, and even across resolution for the same simulation. It is therefore important to develop a galaxy formation model and implementation thereof that produces results well-posed for physical interpretation. Moreover, the high computational cost of these simulations limits the number and resolution of halos available for study, which restricts our insight from a statistical point of view.
The Auriga simulations are a set of cosmological zoom simulations performed with the magneto-hydrodynamics code AREPO and represent our latest efforts at addressing these points. In particular, they offer the following attractive features: 1) excellent convergence properties across resolution, which indicates the results are well-posed for physical interpretation; 2) the high resolution and large simulation sample provide an opportunity to examine the significance and prevalence of a variety of physical processes in one of the most comprehensive galaxy formation model to date.
Gravity and Hydrodynamics
The simulations are performed with accurate and efficient quasi-Lagrangian code AREPO, which follows the coupled evolution of the dark matter and gas components. To follow the magneto-hydrodynamics (MHD), Arepo utilises a dynamic unstructured mesh constructed form a Voronoi tessellation of a set of mesh-generating points (the so-called Voronoi mesh), that allows for a finite-volume discretisation of the Euler equations for magneto-hydrodynamics. The MHD equations are solved with a second order Runge-Kutta integration scheme with high accuracy least square spatial gradient estimators of primitive variables (Pakmor et al.2016a). The gravitational force is calculated with a split Tree-PM approach, in which long-range forces are calculated from a particle-mesh method, and short-range forces are calculated with a hierarchical octree algorithm.
Galaxy formation model
The main features of our galaxy formation model are as follows:
- Primordial metal-line cooling with self-shielding corrections.
- Gas cells with a density greater than 0.13 particles per cubic cm enter the star forming regime, in which the interstellar medium (ISM) is modelled as a two-phase medium composed of cold, dense clouds embedded inside a hot, ambient medium. The ISM is governed by an effective equation of state.
- Star formation occurs stochastically according to a KS-law and a Chabrier initial mass function.
- Stellar evolution, gas recycling and chemical evolution is followed through the distribution of mass and metals to surrounding gas cells at rates corresponding to Sn Ia, SN II and AGB stars. Nine elements (H, He, C, N, O, Ne, Mg, Si and Fe) are followed.
- Stellar feedback is implemented in the form of isotropic winds, which are launched stochastically from SNII sites at a velocity that scales with the local dark matter velocity dispersion. The mass and metal content of the winds is parametrised by the mass and metal loading factors, respectively. The winds are decoupled hydrodynamically until the wind particle enters a gas cell of density lower than a pre-determined threshold density, at which moment the energy (kinetic and thermal), metals, mass and momentum of the wind particle is deposited into the gas cell.
- Black holes are seeded at a mass of solar masses in haloes of masses greater than . Black holes are grown through merging and accretion from nearby gas cells. The accretion rate includes two channels: quasar mode and radio mode. The former is governed by Bondi-Hoyle-Lyttleton accretion, and the latter balances energy losses from the X-ray halo. Both channels of feedback are thermal.
- Magnetic fields are seeded homogeneously at the beginning of the simulations () at a strength of Gauss. A divergence scheme is implemented to ensure .
Initial Conditions
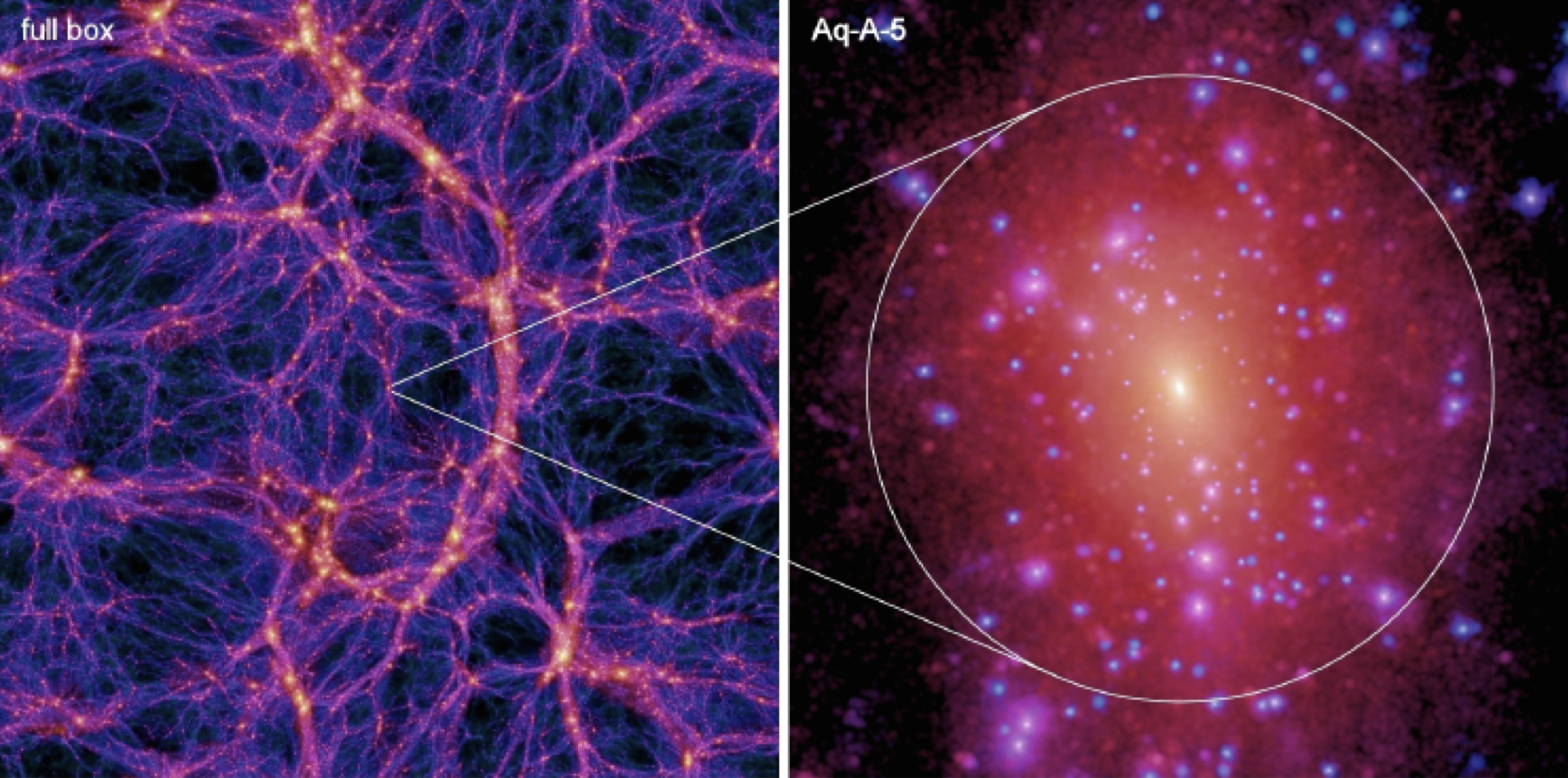
The haloes were selected from a parent dark matter only simulation. The main selection criteria are that the halo mass at redshift zero is in the range , and that the halo satisfy a mild isolation criterion that requires an eligible halo to be a certain distance from a halo of comparable mass. A sample of 174 candidates were selected from the most isolated quartile, and 30 haloes were randomly chosen from this list to form the Auriga haloes.
For a given halo, at redshift zero all particles within a sphere of 4 virial radii were chosen and traced back to their positions at in order to identify the so-called Lagrangian region inside which the halo forms. For the initial conditions, the number of particles is increased in order to refine the resolution in this region, and gas is added by splitting each dark matter particle in mass according to the cosmic baryon fraction.
The table below summarises some of the basic properties of the main suite of standard resolution simulations.
Galaxy properties
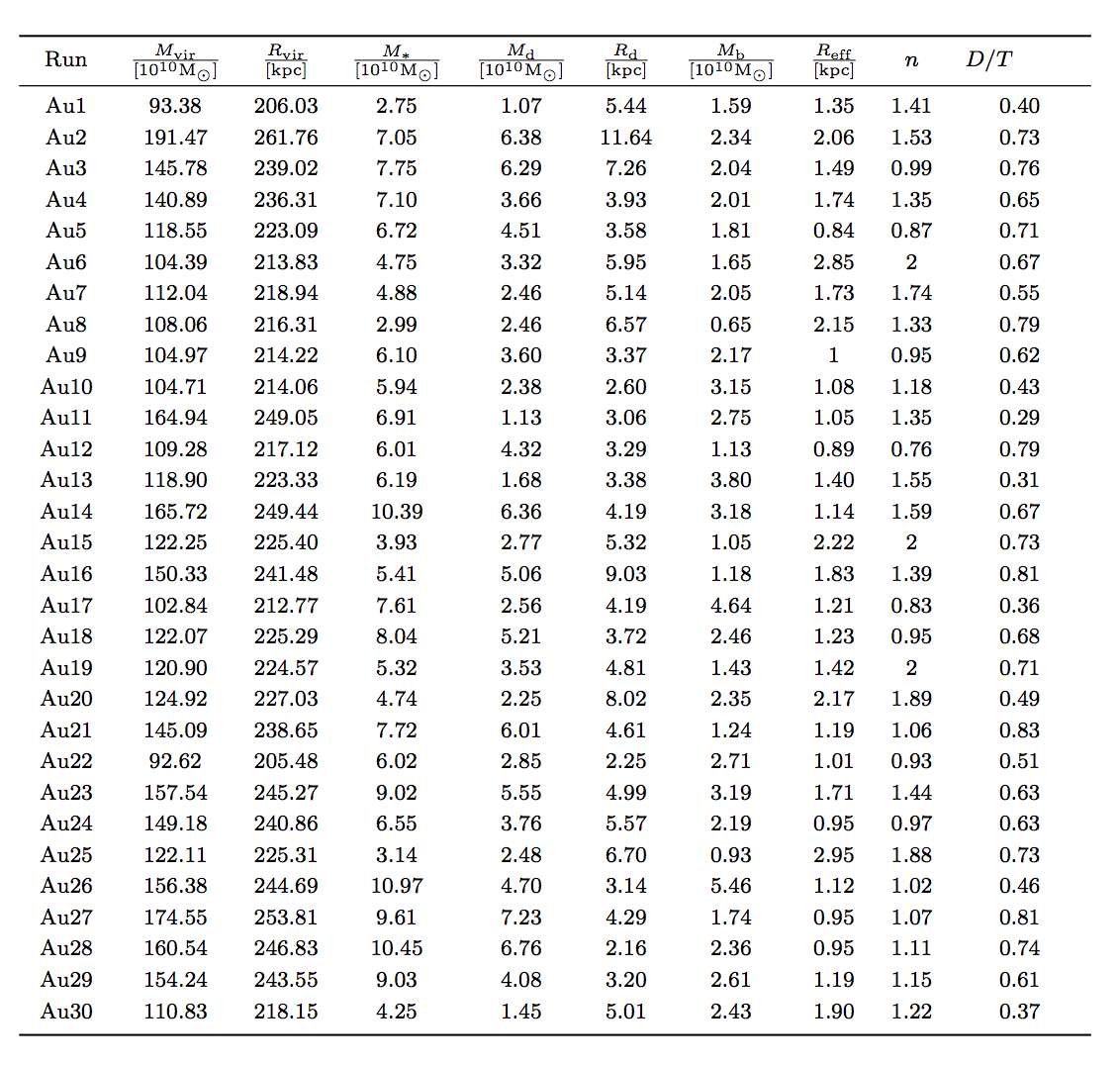
- Halo name
- Virial mass
- Virial radius
- Stellar mass
- Disc mass
- Disc radial scale length
- Bulge mass
- Bulge effective radius
- Sersic index
- Disc to total mass rtaio
The disc and bulge parameters are derived from a simultaneous fit of a Sersic+exponential profile to the surface density profile of the face-on galaxy.