Here, you read more about my research interests, current and past projects including publications.
Research Interests
Understanding the physical processes that form the galaxies and
set their evolution has been one of the central problems in
astronomy. With the advent of advancements in computer simulations
combined with powerful telescopes, we have slowly started to
uncover the hidden mysteries of our vast universe. Recent large
scale cosmological simulations have revealed that the galaxies are
surrounded by a diffuse gaseous halo, known as the circumgalactic medium
(CGM). The interplay between different gas flows in this
invisible medium is perhaps the key to a clear and accurate
picture of the fate of galaxies. In a broader sense, I want to
understand the -
- physical processes that govern the galaxy formation and evolution.
- the complex nature of the gas flows in the CGM and how do they depend on and impact the galaxy properties and its environment.
- methods and techniques required to analyse the large datasets coming from various telescopes.
See my ADS or Google scholar page for publications.
Graduate Projects
In my PhD thesis, I am exploring the nature of the complex gas flows in the galaxies' circumgalactic medium (see Figure) using absorption lines detected in the spectra of background Quasars. My goal is to
constrain the physical properties of these gas flows with the
absorption lines that trace different phases in the CGM. The setup is
the following: when the light emanating from a bright
background source (e.g., quasar) passes through the CGM of a
foreground galaxy, it can get absorbed and cause absorption
dips in the spectrum at redshifts smaller than the quasar
redshift.
The different absorption lines can trace different phases and
physical processes in the CGM and help us understand their
connection to galaxy properties and its environment. For e.g.,
MgII (Mg+) is a tracer of the cold phase (T ~ 104
K) of CGM, while CIV (C3+) traces the warm-hot (T ~
105 K) CGM. In my project, I have explored the
nature and origin of MgII absorbers in the CGM of star-forming
and passive galaxies by characterizing their spatial
distribution. Understanding the connection between this
gas, the galactic properties and its environment does
provide more insights into the physical processes governing
galaxy formation and evolution.
MgII / FeII absorbers in SDSS quasars
In the first paper (Anand et al.
2021), I used the largest quasar catalogue from the
SDSS Data Release 16 (DR16) to search for MgII absorbers
in their spectra. Given large dataset (~ 1 million quasar
spectra), I designed a novel automated pipeline to model the
quasar continuum and search for MgII doublets in their
spectra.
The pipeline uses a dimensional reduction technique called Non-negative
matrix factorization (NMF) , which reduces the quasar
intrinsic emission features into eigenvalues and eigenspectra
to model the quasar continuum. I
developed an automated absorber detection pipeline using a
matched kernel convolution technique and adaptive S/N
criteria. The pipeline can run parallelly on several quasars at once to reduce the absorber search time. The detection
algorithm was run on all quasars from SDSS DR16 and compiled
the largest MgII / FeII catalogue available to date, which
included ~160,000 systems. The absorber catalogue is publicly
available (see below). The figure on the right shows one
example spectra with normalized flux (black) and NMF continuum
(red), along with two MgII absorbers that our pipeline
detects.
The cool CGM of star-forming and passive galaxies
In the same work (Anand et al. 2021), I also connected MgII absorbers with CGM of emission-line galaxies (ELGs or star-forming) and luminous red galaxies (LRGs or passive) from the SDSS DR16 to characterize the properties of cold gas in their CGM. With a very robust statistical analysis, our study implied that cool circumgalactic gas has a different physical origin for star-forming versus quiescent galaxies.
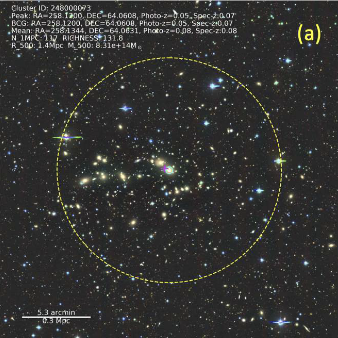
Tracing cool gas in galaxy clusters
In Anand et al. 2022, I extended the MgII absorber study to the galaxy clusters using our MgII absorber catalogue and galaxy clusters from the legacy survey imaging of Dark Energy Spectroscopic Instrument (DESI) data release (DR8). It is one of the most extensive MgII absorber-cluster correlation studies to date, with 160,000 MgII absorbers and 72,000 clusters with spectroscopic redshifts. I characterized the nature and origin of MgII absorbers in galaxy clusters, where most of the intracluster medium (ICM) is mainly filled with hot plasma (T ~ 107 K). Furthermore, I also investigated the connection between MgII absorbers and the member galaxies of the cluster. This analysis has revealed that cool gas in clusters, as traced by MgII absorption, is: (i) associated with satellite galaxies, (ii) dominated by cold gas clouds in the intracluster medium rather than by the interstellar medium of galaxies, and (iii) may originate in part from gas stripped from these cluster satellites in the past.
The multiphase gas in quasar halo
In Anand et al. (in prep.), I am looking into the nature of warm (traced by CIV absorbers) and cool gas (traced by MgII absorbers) in quasar halo. As the physical properties of quasars are very different (due to the presence of central AGN source) from the normal galaxies, the differences must be visible in the properties of gas around them.
Previous Projects
In my master's thesis, I worked on the nature of HI disks
surrounding radio galaxies using the Very Large Array
radio telescope. I also explored the properties of the stellar
atmosphere in Hydrogen deficient
stars during my bachelor's thesis. My goal was to find
the sources of continuum opacity in their atmosphere.
In addition to these extensive projects, I also explored topics in
high energy physics and computational nonlinear dynamics to
broaden my horizon of understanding and develop a richer
appreciation of physics.