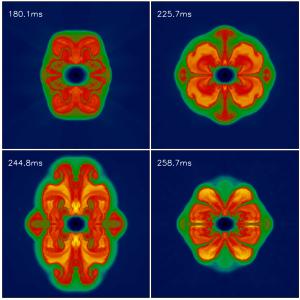 |
Figure 1:
Four snapshots during the simulation of the collapse
of a rotating star with 15 solar masses. The pictures
show violent (convective) mass motions in the region
of neutrino heating around the neutron star
at the center. Between 0.18 seconds (upper left) and
about 0.26 seconds (lower right) after the formation of
the neutron star, the supernova shock front (which is
visible as the discontinuity between blue and green)
reveals huge pulsations and strong deformation.
The displayed region is 620 km wide, the rotation axis
goes vertically through the center (MPEG movie (4.4M)).
|
|
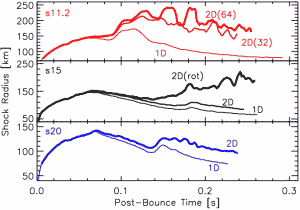 |
Figure 2:
Temporal variations of the mean shock radius for
simulations of three stars with different masses
(from top to bottom: 11.2, 15, and 20 solar masses).
In none of the cases the shock expands farther than
250 kilometers, no explosion occurs. The thin lines
display the results of spherically symmetric
calculations compared to the two-dimensional runs,
which demonstrate the important role of violent
convective mass motions.
|
|
|  |
Stars with more than about ten times the mass of our Sun develop
iron cores at their center which eventually become unstable and
collapse to neutron stars. The interior of neutron stars is denser
than nuclear matter and initially extremely hot. Particle reactions
at such conditions create neutrinos in huge numbers. These
elementary particles have no electric charge and less than one
millionth of the electron mass. They eventually escape from the
dense neutron star, but before leaving the stellar interior they
deposit some of their energy in the still infalling outer layers
of the star. This neutrino heating is believed to cause the
violent disruption of the star in a supernova explosion. Such
catastrophic stellar deaths are not only among the brightest
events in the universe and the birth places of neutron stars
or black holes, they are also the sources of
elements like iron, silicon, and oxygen, which are indispensable
to form rocky planets and human life. Understanding the mechanism of
the explosion is therefore of fundamental importance for a broad
variety of astrophysical problems. (see "How do massive stars explode?")
A group of researchers at the Max-Planck-Institute for Astrophysics
set out to test the current theory with so far unattained precision.
Running a newly developed computer program on Germany's fastest
supercomputer, the IBM "Regatta" system at the Rechenzentrum
of the Max-Planck-Society in Garching,
the group for the first time achieved to describe the
neutrino production and interactions in great detail. The
calculations required each a total of several hundred thousand
trillion (several 10^17) floating point operations, which ranks
them among the most expensive computer simulations ever done.
They also included the effects of stellar rotation and of
violent anisotropic plasma motions. Convective processes in the
supernova core had been recognized to accelerate the energy
transport inside the neutron star and to enhance the deposition
of energy by neutrinos in the outer stellar layers, thus
supporting the explosion of the star.
The outcome of these worldwide most elaborate supernova simulations
was disappointing: No explosions could be obtained. This negative
result shatters the widely accepted view of how the
explosion starts. Theorists have to reconsider their ideas.
What is missing in the current models? Do we understand the
properties of the nuclear medium in the neutron star well enough?
Do we really know how neutrinos interact with particles in the
very dense plasma? Are three-dimensional effects important and
do the current two-dimensional simulations therefore miss crucial
physics? Can magnetic fields be safely ignored as in the models
so far?
The latest generation of accurate supernova models therefore
sheds new light on these open questions of supernova
theory. They will keep astrophysicists, nuclear physicists and
particle physicists busy for many years to come.
Robert Buras, Konstantinos Kifonidis, Markus Rampp and Hans-Thomas Janka
Further information:
- R. Buras, M. Rampp, H.-Th. Janka, and K. Kifonidis:
"Improved Models of Stellar Core Collapse and Still no
Explosions: What is Missing?", Phys. Rev. Letters, in press, astro-ph/0303171,
http://xxx.uni-augsburg.de/abs/astro-ph/0303171
- H.-Th. Janka, K. Kifonidis, and E. Müller:
"Entdeckungsreise mit dem Computer:
Dem Rätsel der Supernovae auf der Spur"
http://www.mpa-garching.mpg.de/POPULAR/suw.html
- H.-Th. Janka and E. Müller:
"Supernovaexplosionen massereicher Sterne",
Physik in unserer Zeit 32, p. 202--211 (2001)
http://www.mpa-garching.mpg.de/POPULAR/phiuz_www.pdf
- "Supernova", Film:
"Cosmic Cinema", Webpage of the Max-Planck-Institute for
Astrophysics, http://www.MPA-Garching.MPG.DE/~museum/museum/index.html
- Terascale Supernova Initiative:
http://www.phy.ornl.gov/tsi/
- SciDAC Supernova Science Center:
http://www.supersci.org/
|