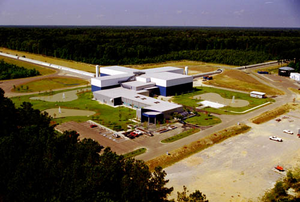 |
Fig. 1:
Aerial view of the
LIGO,
gravitational wave detector at Livingston, U.S.A.; the
two interferometer arms extend to a length of 4 km. Similar detectors
are operating in Hanford, U.S.A.
( LIGO),
in Pisa, Italy
( VIRGO),
in Hannover, Germany
( GEO 600),
and in Tokyo, Japan
( TAMA 300).
Picture from LIGO Livingstone, used by permission.
|
 |
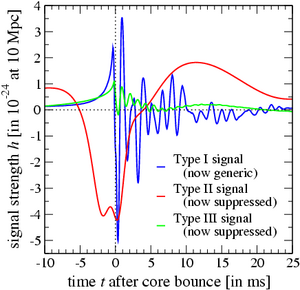 |
Fig. 2:
Generic gravitational wave signals from the collapse of rotating
stellar cores. In the new simulations with the more realistic initial
models and an improved matter model, only Type I signal templates
(blue curve) are found. The Type II (red curve) and III
(green curve) signals known from previous simpler models are
completely suppressed.
|
 |
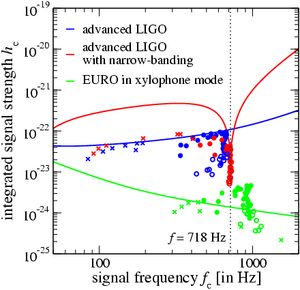 |
Fig. 3:
Detection prospects for gravitational wave signals from extragalactic
supernovae. For the proposed regular advanced LIGO detector all signals
(blue points) from an event in the Andromeda galaxy lie below the
sensitivity threshold (blue curve). A narrow-banded advanced LIGO
detector could exploit that the new signal templates are now generic
(with many of them lining up at one frequency like pearls on a string;
red points), and measure some of them (red curve). The (again
broad-banded) future EURO detector is sensitive enough to have
even many of the signals coming from the Virgo cluster of galaxies
(green points) above its detection threshold (green curve). The
locations of the signals in this graph depend on the detector
characteristics (as color-coded).
|
|  |
While light or sound waves propagate through space and time,
gravitational waves are propagating ripples of space and time
itself. Such distortions of spacetime are generated by
catastrophic astrophysical phenomena involving extremely compact
objects (like e.g. colliding and merging black holes or neutron stars,
or the collapsing core of a star in a supernova event).
Despite its origin in such a violent environment, a gravitational
wave arriving on Earth will have a relative signal strength of at most
10-20, which is only one part in 100,000,000,000,000,000,000.
This corresponds to a spatial displacement of only about 1/100th of
the size of an atomic nucleus in a 100 km long measuring rod!
Although gravitational waves were predicted by Einstein already at the
beginning of the 20th century, only now we have the technology to
venture the first successful detection. Currently, five
kilometer-class Laser interferometer detectors are actively scanning
the sky for incoming gravitational waves from both Galactic and
extragalactic events (see Fig. 1). They are supported by resonant
detectors, which in contrast to the current interferometers have
their optimal sensitivity only in a very narrow frequency band.
In the forseeable future, the existing experiments will be upgraded
for better sensitivity, new already planned detectors are scheduled to be built, and
the space-based satellite LISA will complement the detectors on Earth.
A successful direct detection will not only unequivocally prove
Einstein's bold prediction, but even more interestingly, will open a
completely new "window" onto the universe. By routinely observing
gravitational waves, astrophysicists will gain new and otherwise entirely
unattainable insights into such fascinating objects like black holes,
the enigmatic cosmic gamma ray bursts, or the driving engines behind
stellar supernova explosions.
One example of a promising source of gravitational waves is the
gravitational collapse of the rotating core of a massive star to a
neutron star and the subsequent explosion of the star in a spectacular
supernova event: During the collapse, within fractions of a second, a
mass larger than that of our sun is compressed to densities exceeding
100 millions of tons per cubic centimeter, until the contraction is
stopped by the core bounce. However, so far the search for
gravitational waves from a supernova was impeded by the rather crude
knowledge of the expected burst signal from the core bounce.
A first approach to produce templates of gravitational wave signals
from stars dying as a supernova was already successfully undertaken
several years ago at the Max Planck Institute for Astrophysics
(see Research Highlight November 2001).
However, these templates predicted a large variability of the way
the collapse and bounce proceeds. The corresponding uncertainty of
the wave signal hampered an application of the most efficient
available filters in the detector signal analysis.
Greatly improving on these previous results, again scientists from the Max
Planck Institute for Astrophysics in cooperation with Christian D. Ott
(previously Max Planck Institute for Gravitational Physics,
Golm, Germany; now
Department of Astronomy and Steward Observatory,
University of Arizona,
U.S.A.) and supported by Ian Hawke
( School of Mathematics,
University of Southampton,
U.K.) as well as by Erik Schnetter and Burkhard Zink (both
Center for Computation & Technology,
Louisiana State University,
U.S.A.)
have now accomplished new multi-dimensional supercomputer simulations of
many rotating stellar core collapse models. In contrast to the old
simpler computations, now much better initial models for the
pre-collapse stellar cores were used, a more realistic microphysical
description of matter was implemented, and the effects of neutrinos
during the contraction phase were taken into account.
Most interestingly, with all these important improvements taken into
account, the previously obtained diversity of the
gravitational wave signals disappears, with only one
signal type surviving (see Fig. 2). This type is known as
Type I and is characterized by a positive pre-bounce signal
rise and a large negative spike during bounce, followed by a ring-down
phase during which the signal slowly dies off.
The finding that for improved models the gravitational wave
templates become more generic has important implications for a
possible detection of such an event. Obviously, with better knowledge
of the expected signal and more robust predictions, fine-tuned data
analysis methods can be applied to extract such signals from the
data streams measured in the detectors. More importantly, as the
frequencies of the signal templates cluster in a very narrow
range, the interferometer detectors themselves can be fine-tuned to
search predominantly in a narrow frequency band (see Fig. 3). In addition,
resonant detectors, which are already narrow-banded by construction,
can very effectively join the search.
With such techniques and a combined effort of several detectors,
the detection range can probably be extended far beyond our Galaxy in
the near future. This greatly increases the prospects for a signal
detection, as otherwise hopes would solely rest on the rather rare
event of a supernova in our own Galaxy.
H. Dimmelmeier, H.-T. Janka, A. Marek, E. Müller
Publication
C.D. Ott, H. Dimmelmeier, A. Marek, H.-T. Janka,
I. Hawke, B. Zink, and E. Schnetter,
"3D Collapse of Rotating Stellar Iron Cores in General Relativity with Microphysics",
Physical Review Letters, submitted;
astro-ph/0609819
H. Dimmelmeier, C.D. Ott, H.-T. Janka, A. Marek,
and E. Müller,
"Generic Gravitational Wave Signals from the Collapse of Rotating Stellar Iron Cores",
Physical Review Letters, submitted;
astro-ph/0702305
C.D. Ott, H. Dimmelmeier, A. Marek, H.-T. Janka,
B. Zink, I. Hawke, and E. Schnetter,
"Rotating Collapse of Stellar Iron Cores in General Relativity",
Proceedings of the New Frontiers in Numerical Relativity Conference, AEI Golm, Germany, 2007;
astro-ph/0612638
|