|  |
During their lifetimes, stars "burn" light elements such as hydrogen into heavier
ones by nuclear fusion. This process produces energy until, at the end, an iron
core is formed. Since iron has the largest binding energy of all nuclei, no
heavier elements can be produced in fusion reactions and nuclear burning ceases.
However, the iron core continues to grow by fusion processes at its surface. At
this stage, gravity is balanced by the quantum mechanical pressure of the
electrons. Similar to a white dwarf star, there is a critical mass above which
the iron core can no longer resist the pull of gravity and collapses. Under
appropriate conditions, this results in a powerful stellar explosion: a
supernova.
Already in the middle of the past century, first theories proposed the origin of
the supernova energy: Because of the extreme gravitational force, the core
collapses within fractions of a second to produce a neutron star. Gravitational
binding energy is released and transported outwards by a shock front, but gets
quickly absorbed by the outer layers of the iron core. To actually trigger the
explosion, an additional effect is required: heating by neutrinos (see Highlight 2001). These elementary particles are generated in vast numbers in the
new-born neutron star and propagate outwards relatively freely, once they are
outside the neutron star's surface. Therefore they can extract energy from the
so-called "cooling layer" and deposit this energy at greater distances from the
neutron star where they are re-absorbed and thus heat the plasma in the
so-called "heating layer" behind the shock wave. If the amount of deposited
energy is large enough, the shock is pushed outwards, which eventually disrupts
the star in a supernova. At least that is how the theory goes.
The process to confirm this paradigm in detailed physical models, however, has
been long: In the 1980s, the first star "exploded" in a computer, but only in
spherically symmetric (i.e. one-dimensional) models and with some special
assumptions to simplify the description of the physics involved. But the
observation of supernova 1987A showed that multi-dimensional effects play an
important role during the explosion. The shells surrounding the neutron star are
mixed by convection, which further supports neutrino heating. After a few
decades, scientists could confirm the basic functioning of the neutrino
mechanism with two-dimensional models (see Press Release 2009). Still, the
forced rotational symmetry about an arbitrary axis severely restricts motions of
the stellar plasma. In addition, turbulent flows behave differently under these
symmetry assumptions compared to three dimensions. It is therefore necessary to
perform three-dimensional calculations to model all processes during the
supernova correctly.
So far, simulations have not yielded successful explosions in three dimensions ( Press Release 2013
und Highlight 2014). But now, the scientists obtained
their long desired result: the first successful, neutrino-driven explosion of a
star with an initial mass of 9.6 solar masses in a three-dimensional,
self-consistent simulation (see Fig. 1). The challenge was to describe the
neutrinos as correctly as possible, so that the resulting complex calculation
kept even supercomputers busy for a few months. The new method provides the
currently most complete description of how neutrinos interact with matter in a
supernova calculation. In particular, there is an open, controversial question
whether three-dimensional turbulence in the neutrino heated plasma helps or
hinders the explosion.
In this case, the answer is definitely yes: three-dimensional turbulence leads to about 10% higher explosion
energy. Turbulent effects in the heating layer change the flow of stellar material into the cooling layer, which means that the
temperature in this region remains lower. As the cooling by neutrinos strongly
depends on temperature, the energy loss by neutrino emission decreases at lower
temperature and the explosion gets stronger. However, it is difficult to predict
whether this phenomenon could play an equally important role for even more
massive stars. To answer this question, the scientists need further simulations.
They also plan to calculate the explosion with even higher resolution to better
resolve turbulence and investigate it on smaller scales. Another important
question is whether the star might have been asymmetric before collapse and how
this would affect the explosion. So even with this significant milestone, the
astrophysicists still have some way to go.
Tobias Melson, Hans-Thomas Janka
Public outreach: Hannelore Hämmerle
Publication:
T. Melson, H.-T. Janka, & A. Marek, Neutrino-driven supernova of a low-mass
iron-core progenitor boosted by three-dimensional turbulent convection,
Astrophysical Journal Letters, 801, L24 (2015)
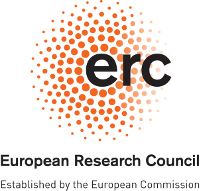 |
This project has been supported by the European Research Council
through grant ERC-AdG No. 341157-COCO2CASA. |
Furthermore, the authors acknowledge funding by the Deutsche
Forschungsgemeinschaft through the Excellence Cluster EXC 153 "Origin
and Structure of the Universe" and computing time provided by the
European PRACE Initiative on SuperMUC (GCS@LRZ, Germany) and Curie
(GENCI@CEA, France).
|