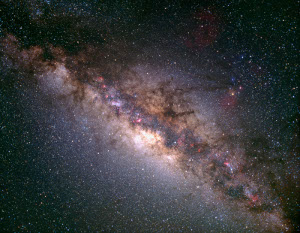 |
Fig. 1:
The Milky Way contains about 300 billion stars. The galactic disk,
which is partly hidden behind gas and dust clouds, is surrounded by a
spherical halo of old stars and stellar clusters.
Image: Wei-Hao Wang
|
 |
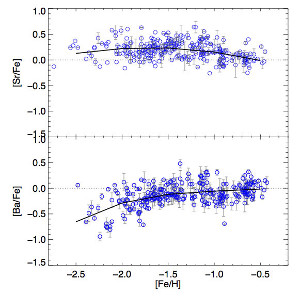 |
Fig. 2:
The new barium (Ba) and strontium (Sr) abundances for the halo and
disk stars as a function of metallicity. The measurements show the
difference between the ratio of each element to iron in a star and
that of the Sun (i.e., a value of zero means that the star has the
same abundance ratio as the Sun). Disc stars with low metallicity
(-1.5 < [Fe/H] -0.5) show solar barium abundances, whereas for halo
stars the barium abundance is consistent with that predicted from the
r-process in supernova explosions. The strontium abundances, however,
do not agree with predictions from standard nucleosynthesis models.
|
 |
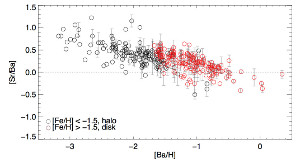 |
Fig. 3:
Relative abundance of strontium and barium, where disk and halo stars
are indicated by red and black circles. Conventional nucleosynthesis
scenarios would give a slightly negative value for [Sr/Ba], whereas
the observed value is clearly positive, especially for the stars with
very low metallicity, [Fe/H] < -1.5.
|
|  |
In theory, the nucleosynthesis of elements heavier than iron is
thought to be well understood. Most of them are formed in the
so-called slow (s) or rapid (r) neutron capture processes on lighter
seed nuclei. The s-process elements are mainly produced during the
late stages of evolution of low-mass stars; a small fraction of these
elements originates from massive stars, which explode as core-collapse
supernovae.
However, predictions of the stellar models are not fully supported by
observations. The observed s-process abundances in the Sun and
metal-poor stars, tracing the composition of the interstellar matter
in different stages of Galactic evolution, need to be explained by
alternative nucleosynthesis scenarios.
Strontium (Sr) and barium (Ba) with magic neutron numbers of 50 and
82, respectively, are the most abundant s-process elements. This is a
direct consequence of their nuclear properties, namely very small
neutron capture cross-sections. In addition, their strongest
absorption lines are in the optical part of a spectrum, accessible
with ground-based telescopes, and can be measured even in most
metal-poor and distant Galactic halo stars.
The most difficult part, in fact, is to compute the abundance from the
observed data points: line profiles are very sensitive to the
treatment of radiative transfer in stellar atmosphere models. So far,
most observational studies in the literature relied on
highly-simplified models, neglecting the important influence of
non-local thermodynamic equilibrium (non-LTE) effects on element
abundances.
Scientists at MPA have now performed, for the first time, a consistent
non-LTE spectroscopic analysis of a large sample of metal-poor stars,
which belong to various Galactic populations (halo, thick and thin
disks). Non-LTE effects were taken into account both in the
determination of the basic stellar parameters and the element
abundances (see Fig. 2), using new quantum-mechanical atomic data.
The disk stars show barium abundances in agreement with scaled solar
abundances. This can be fully explained by models of nucleosynthesis
in low-mass stars. The halo stars exhibit a strong deficiency of
barium compared to iron. This is again consistent with stellar models,
which predict that only a small range of massive stars contribute to
producing barium via the r-process, whereas iron is made in supernovae
explosions of stars with different initial masses.
The strontium abundances, however, increase with decreasing
metallicity of a star eventually flattening off at the lowest
metallicities. This conflicts with predictions from stellar
models. What process could have produced strontium in the early
Galaxy? Furthermore, what process over-produced strontium compared to
barium at the time when the oldest and very metal-poor stars were
born? Summing up all known contributions from both r-process and
s-process gives a strontium-to-barium ratio, which is much lower than
the one observed for stars with low metallicity (see Fig. 3).
A few non-standard, exotic nucleosynthesis scenarios, such as the
so-called Light Element Primary Process or low-mass electron-capture
supernovae, might have the potential to explain these observations.
Gregory Ruchti, Maria Bergemann
References
Travaglio, C., Gallino, R., Arnone, E., et al.,
"Galactic Evolution of Sr, Y, and Zr: A Multiplicity of Nucleosynthetic Processes",
2004, ApJ, 601, 864
Wanajo, S., Janka, H.-T., Mueller, B.,
"Electron-capture Supernovae as The Origin of Elements Beyond Iron",
2011, ApJL, 726, L15
Ruchti, G. and Bergemann, M.,
"New NLTE Results for Neutron-Capture Elements in Metal-Poor Milky Way Field Stars",
2012, MNRAS, in prep.
|