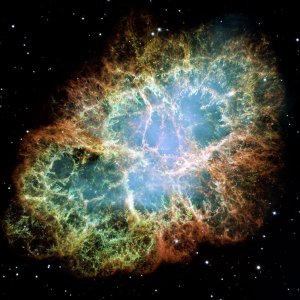 |
Fig. 1:
Fig. 1: The Crab Nebula with the Crab pulsar, the gaseous
and compact remnants of a supernova explosion that occurred
in the year 1054 A.D. Relativistic particles, which are accelerated
by the pulsar, cause the bluish glowing of the gas even 950
years after the explosion. The outer filaments consist of mostly
hydrogen and helium of the disrupted star.
Source: www.spacetelescope.org
Credit: NASA, ESA and Allison Loll/Jeff Hester (Arizona State University)
Acknowledgement: Davide De Martin ( www.skyfactory.org)
|
 |
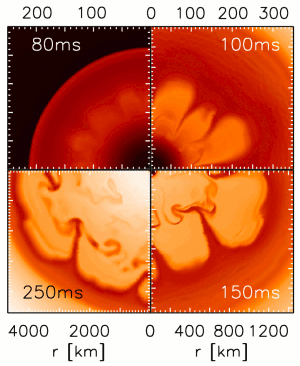 |
Fig. 2:
Fig. 2: Snapshots from a two-dimensional computer simulation of the
onset of the supernova explosion of a star with 8--10 solar masses.
The displayed times are 0.08, 0.1, 0.15, and 0.25 seconds after the
creation of the supernova shock (clockwise, beginning with the upper left
panel). One can see rising bubbles of neutrino-heated matter, separated by
narrow down-flows of cooler gas. The bubbles expand away from the nascent
neutron star at the center and accelerate the stellar explosion.
Note that the radial scale (in kilometers)
changes by a factor of 20 from the upper left to the lower left
panel. These anisotropies are the seeds of the asymmetries seen
in the Crab remnant 950 years after the supernova event.
There is also a
movie (MPEG,32MB)
of this simulation.
Credit: Max Planck Institute for Astrophysics
|
 |
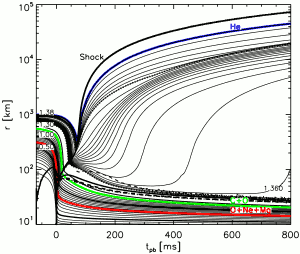 |
Fig. 3:
Computer simulation of the collapsing and exploding
core of a star with 8--10 solar masses. The lines follow the radial
positions of selected shells in the star as time evolves.
The shock wave of the explosion is marked by the bold, rising, black
solid curve. One can see a bifurcation developing between the
matter that forms the central neutron star and the ejected gas that
is driven outward by the heating of neutrinos. Colored lines indicate
composition interfaces in the dying star and show that only little
carbon and oxygen are thrown out in the explosion.
Credit: Max Planck Institute for Astrophysics
|
|  |
When Chinese and Arab astronomers watched the sky in the
spring of the year 1054 A.D., they discovered a new
star in the constellation of Taurus. According to their
historical records, the "guest star" became brighter
during several weeks and could be observed by July
for 23 days even in the daytime. It remained
visible to the naked eye for about two years.
Now we know that they observed the birth of the Crab Nebula
by a gigantic supernova explosion. After millions of years
of quiet evolution, a massive star had exhausted
its supply of nuclear fuel, whose burning had provided the
energy and pressure to stabilize the star against the pull
of its own gravity. When the nuclear flame in its center
died, the stellar core collapsed within fractions of a second
to a neutron star, a compact object with more mass than the Sun
but a diameter of only 20 kilometers. This neutron star is
visible as the famous pulsar in the Crab Nebula, which
sends periodic pulses of radiation as it spins around its
axis 33 times per second.
Most of the star, however, was ejected in a violent explosion
with an energy roughly equal to what the Sun has radiated
in 5 billion years of its life. The hot stellar debris flashed
up as the new star reported by the Chinese and Arab astronomers,
and is nowadays visible as the filamentary gas cloud of the
Crab Nebula measuring six light-years across and still expanding
with a velocity of 1500 kilometers per second (Fig. 1). It contains
not only the chemical elements which the star has built up in a
sequence of nuclear burning stages — first fusing hydrogen to
helium, then helium to carbon, and then carbon to neon, magnesium,
and oxygen — but also material like radioactive nickel,
which was freshly assembled during the explosion.
The helium richness of the nebula and the low abundances
of carbon and oxygen were interpreted as hints that the exploding
star had a mass of only about 8 to 10 solar masses, just
sufficient to end its life as a supernova.
But how did the star blow up? What was the reason why the star was
disrupted? A group of researchers of the Max Planck Institute for
Astrophysics is convinced that they have now found the answer of this
long standing conundrum. Their refined computer simulations reveal
that neutrinos are the driving force behind the explosion. These
elementary particles are produced in huge numbers in the very hot and
extremely dense interior of the newly formed neutron star, mainly by
reactions of electrons and positrons with protons and neutrons, the
constituents of atomic nuclei. Having made their way to the surface of
the neutron star, most of these neutrinos stream off and carry away
more than 99 percent of the energy liberated during the neutron star
formation. Less than one percent of the neutrinos, however, is
captured in the stellar gas surrounding the neutron star before being
able to escape. The energy transfer by these neutrinos heats the gas
and makes it boiling like the fluid in a pressure cooker (Fig. 2). The
rising pressure finally accelerates the overlying stellar material and
leads to the outburst of the supernova (see current
research, february 2001).
Although this theory for the onset of the explosion is 25 years old,
proving its viability with detailed computer models turned out to be
extremely difficult (see current
research, june 2003). Now at least for stars near the lower end
of the mass range of supernova progenitors the models lend support to
the theoretical idea. "With our refined description of how neutrinos
are created and interact in the matter in the supernova core, we were
able to confirm that neutrino heating indeed can drive healthy
explosions of stars like the one whose relics form the Crab Nebula",
says Francisco Kitaura of the team of astrophysicists who performed
the computer simulations. The new models agree nicely with
observations that the energy of the Crab explosion was only about one
tenth of that of a typical supernova. Different from previous
simulations they also predict only small amounts of ejected carbon,
oxygen, and nickel (Fig. 3). Moreover, the strong enrichment of the
chemical composition of the remnant with exotic elements is absent and
thus a conflict of the older models with the observed abundances of
rare elements in the Milky Way Galaxy. Since the disrupted star had a
rather low mass and the explosion was sub-energetic with little
production of radioactive material, other Crab-like supernovae must be
expected to be fairly dim and therefore difficult to discover at great
distances, although they could account for one third of all
supernovae.
"Our computer models suggest that the Crab supernova was such a
tremendously bright event only because it was just 6300 light-years
from Earth," explains Wolfgang Hillebrandt, the leader of the research
team. "Compared to other supernovae it actually was a fairly
unspectacular case. Our computer models will tell us what we have
to look out for in order to identify more such cases."
F.S. Kitaura, H.-Th. Janka, R. Buras, A. Marek, W. Hillebrandt
Reference:
F.S. Kitaura, H.-Th. Janka, and W. Hillebrandt, Explosions of
O-Ne-Mg cores, the Crab supernova, and subluminous type II-P
supernovae, Astronomy and Astrophysics, 450 (2006) 345-350
Further information:
Hans-Thomas Janka, thj mpa-garching.mpg.de
|