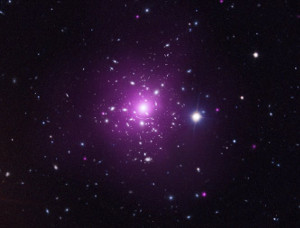 |
Figure 1:
A composite optical and X-ray image of Abell 383, one of the 7 relaxed rich clusters considered in the study
by Newman et al. 2013a,b. This image shows the X-ray emission of the hot electron gas in the cluster (in purple),
its member galaxies and its central Brightest Cluster Galaxy which exhibits an extended diffuse envelope of stars
around it.
Credits: X-ray: NASA/CXC/Caltech/A.Newman et al/Tel Aviv/A.Morandi & M.Limousin;
Optical: NASA/STScI, ESO/VLT, SDSS
|
 |
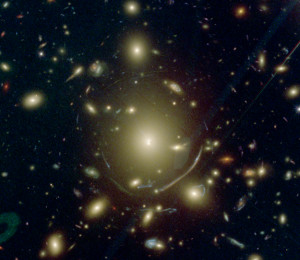 |
Figure 2:
A zoom on the BCG in Abell 383 taken with the Hubble Space Telescope. The
central BCG is surrounded by an extended envelope of stars and the numerous
distorted images around it are background galaxies which are getting lensed
by the cluster. Because of their high masses, galaxy clusters can act as
gravitational lenses: the background galaxies close to the line of sight of
the cluster get multiply imaged or distorted into large arcs like the one
visible south of the BCG. Some of the cluster galaxies (e.g. the bright
elliptical galaxy one on the south-east of the BCG) act as additional lenses
which further distort some of the multiple images.
Credits: NASA/STScI
|
 |
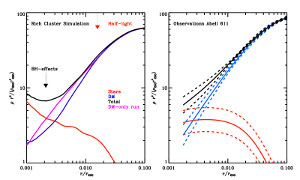 |
Figure 3:
Density profiles of simulated and real clusters.
Left Panel: Density profile for one of the re-simulated galaxy cluster.
The black, red and blue lines
represent the distribution of total (stars+dark matter), dark matter and
stellar mass. The magenta line corresponds to the distribution of matter
in a dark-matter-only run of the cluster (where the contribution of stars
in galaxies was completely neglected). The total mass profile as a whole
is very similar to the dark-matter-only run except where the density of
stars overtakes that of the dark matter. The final dark matter profile on
the other hand is shallower than the original dark-matter-only run already
at the half-light radius of the BCG marked by the red arrow. The black
arrow shows the radius where effects from black hole mergers would
significantly affect the distribution of stars and dark matter in the BCG
core.
Right Panel: Density profile for one of the clusters in the Newman et al.
(2013) sample, Abell 611. Black, red and blue lines represent the
contributions from total, stellar and dark matter respectively. The dashed
lines mark the 1-sigma error on the modelling. The mass distribution in
this cluster is quite similar to one of the simulated clusters in the left
panel.
Credits: Laporte & White 2014
|
|  |
The Cosmic Microwave Background provides important information on how dark
matter was distributed in the early Universe. Cosmological N-body simulations
can be used to follow this distribution as it evolves forward in time,
ultimately giving rise to today's cosmic web, made up of voids, filaments and
the halos in which the galaxies live. It is an important task to characterise,
both theoretically and observationally, the internal structure of these halos,
since this constrains both the nature of the dark matter particle and the way
galaxies form and evolve. Already in the 1990s, cosmological N-body simulations
were able to characterise the density profiles of dark matter halos, showing
that, to a good approximation, these have a universal shape from the scales of
dwarf galaxies to those of galaxy clusters. The physical origin of this
universal profile remains a mystery to this day. An important task in modern
astronomy is to infer the distribution of dark matter in galaxies in order to
test this prediction of the standard LCDM paradigm for halo structure.
Galaxy clusters are objects of prime interest to study dark matter because they
give astronomers the largest number of independent probes of halo structure
(stellar kinematics, strong gravitational lensing, weak gravitational lensing,
X-ray emission from hot gas, galaxy motions). This helps considerably in
obtaining robust and precise results which can put firm constraints on total
mass profiles. Recent observations of galaxy clusters and of their central
galaxies (Brightest Cluster Galaxies or BCGs) have combined a number of probes,
revealing that the clusters' total density profiles are well described by the
"universal" profile found in cosmological dark-matter-only simulations. However,
their dark matter profiles are systematically shallower in the innermost regions
(well inside the visible BCG).
As gas cools and condenses near the centre of a dark matter halo and begins to
form stars, simple arguments suggest the dark matter should be pulled inwards,
thus steepening its density profile. While this appears to contradict the
observations, this is not the full story for BCGs because their growth can be
more complicated than that of more typical galaxies. It was proposed in the
1970s that BCGs may grow through multiple mergers of preformed galaxies which
will occur preferentially at the centres of clusters. This suggestion seems to
hold up according to current detailed simulations of the formation of galaxies
and clusters in the LCDM paradigm. However, previous work did not investigate
whether this picture could explain the observed structural evolution of BCGs in
detail (e.g. their stellar masses, sizes, shapes, surface brightness profiles
and dark matter content, all as a function of redshift). A year ago, a team of
scientists at the MPA and the National Astronomical Observatories in China have
provided further support for this formation channel by comparing observations at
low and high redshift with sophisticated methods for "painting" the stars onto
cosmological dark matter N-body simulations of galaxy cluster formation.
More recently, MPA scientists conducted N-body simulations which explicitly and
self-consistently followed the evolution of both stars and dark matter in
clusters. These high-resolution simulations began with a dark matter
distribution consistent with LCDM expectations and a galaxy population
consistent with that observed in the z~2 universe (about 3 billion years after
the Big Bang) and they followed evolution down to the present day. This required
a new scheme to insert equilibrium galaxies of a prescribed structure into dark
matter halos that had already formed in a cosmological simulation, while
mimicking the contraction of the dark-matter halos induced by baryon
condensation at their centres.
While the earlier conclusions on BCG evolution held up, the new simulations
showed that the central mass re-distributes itself significantly as mergers
proceed. By the present day, the mixture of dark and stellar matter in the BCGs
had the same total mass density profiles as in test simulations which included
dark matter alone. This demonstrated that evolution tends to drive the total
mass density profile (stars and dark matter) towards the "universal" shape.
Since the stars contribute most of the mass near the middle of the final BCGs,
this meant that their dark matter density profiles were actually less centrally
concentrated than in the dark-matter-only simulations, even though they started
out more concentrated in the initial galaxies. As a result, the simulated BCGs
appear to have dark matter profiles consistent with those inferred
observationally.
The simulated BCGs typically experienced 6 or 7 mergers which, in real galaxies,
would be accompanied by a merger of the central supermassive black holes. Such
mergers pump energy into the innermost regions, causing the stars and dark
matter to move outwards. Estimates of the size of this effect based on the
simulations suggest that it might explain the large stellar cores often observed
in BCGs. So far, the effects of supermassive black holes in BCGs cannot be
directly simulated in a full cosmological context, so the current simulations
offer realistic initial conditions for simplified numerical studies of
supermassive black hole merging in the central regions of BCGs.
This study suggests that observations of the mass distribution in the centres of
galaxy clusters can be understood if BCG evolution is primarily driven by
dissipationless mergers. Within the standard LCDM paradigm, such an evolutionary
path naturally explains a total density profile similar to those found in
dark-matter-only simulations, together with a shallower dark matter density
profile. There seems no need to appeal to the more radical explanations proposed
in some recent papers such as new physics in the dark matter sector or dynamical
effects driven by star and black hole formation which are much more violent than
any observed.
Chervin Laporte and Simon White
References:
Laporte C. F. P., White S. D. M., Naab T., Gao L. 2013, MNRAS, 435, 901
Laporte & White 2014, http://arxiv.org/abs/1409.1924
Newman 2013a, ApJ, 765, 24
Newman 2013b, ApJ, 765, 25
|