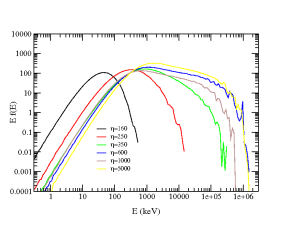 |
Fig. 1:
The simulated spectrum of radiation expected from a magnetically powered
GRB. One important quantity
of the flow is the luminosity-to-mass-flux ratio η; a measure of its
"cleanness". For high values of η the spectra peak at
around 1000 keV. The flat tail above this peak results from
scattering of photons off hot electrons in the flow.
For low η, the magnetic annihilation stops below the
photosphere. The resulting spectrum is then quasi-thermal.
|
 |
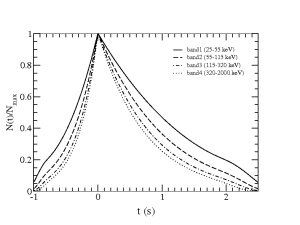 |
Fig. 2:
Example of how observations can tell us something on the
properties of the fast moving material. A gamma-ray-burst light curve
consists of many pulses. Their width decreases with photon energy.
Observations also show that the peak of the radiation spectrum increases with the
luminosity of the burst. Both of these behaviors are reproduced by the
theoretical calculations if the most powerful flows are also the "cleaner" ones.
The plot shows the predicted narrowing of the pulses with increasing
photon energy.
|
|  |
Gamma-ray bursts are detected by satellites about once a day.
The gamma-ray emission lasts from milliseconds to
many minutes and is followed by the "afterglow" emission at lower
energies (X-rays, ultra-violet, optical, infra-red, radio) before they
dissappear for ever.
The majority of observed GRBs appear to be the end result of
the evolution of very massive stars (40 times more massive than our sun, or more)
when the core of the star collapses into a black hole. A specific subclass
of GRBs (the shorter duration bursts) appears to be due to another process, possibly
the collision of two neutron stars orbiting each other.
It has been known for many years that ejection of matter at
velocities very close to the speed of light is necessary
to explain the burst of gamma rays. The acceleration
of matter to these high speeds and the emission mechanisms responsible for
the burst are still somewhat of a mystery. Theoretical studies now show that magnetic
fields may well be the key element for the explanation of gamma-ray bursts
(
Research Highlight February 2002).
The annihilation of magnetic field lines
causes not only the strong acceleration of material, but also heats it up
so that it can glow.
D. Giannios and H. Spruit at the Max Planck Institute for Astrophysics have
looked more closely how radiation interacts with matter in these strongly
magnetized flows. Their study points towards a specific distance from the
explosion center as the most promising location to produce the burst.
This is the distance where radiation stops interacting with matter (the so
called photosphere). At this radius, the energy released by magnetic
annihilation heats the flow. Radiation that is carried with the flow is scattered
by the hot material and gains energy. Numerical simulations show
that the spectrum of radiation from this hot flow is very
similar to the observed gamma-ray spectra.
The calculations directly connect the properties
of the fast moving material to the emitted spectrum. This is a very interesting result since
the study of the observed properties of the burst can be used to make
inferences on the how the ejection of the flow actually takes place.
There are observations of thousands of bursts that can be used to
shed light on this poorly understood phase of gamma-ray bursts.
Dimitrios Giannios
Publication
D. Giannios,
"Prompt emission spectra from the photosphere of a GRB",
A & A, 457, 763(2006);
D. Giannios and H. C. Spruit,
"Spectral and timing properties of a dissipative GRB photosphere",
A & A, in press;
astro-ph/0611385
Contact
Dr. Dimitrios Giannios, Max Planck Institute for Astrophysics,
Tel. 089/30000 2269, Email: giannios mpa-garching.mpg.de
|