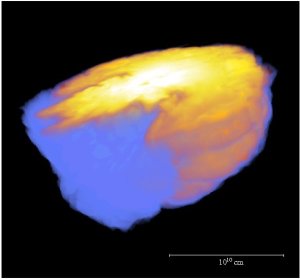 |
Fig. 1:
Slice through the asymmetric composition resulting from a
three-dimensional thermonuclear supernova simulation. Yellow/white
indicates nickel while unburned material is shown in blue.
|
 |
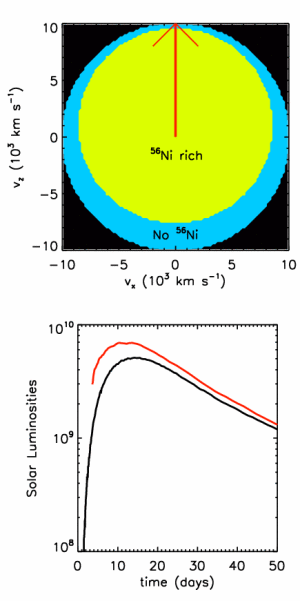 |
Fig. 2:
Simplified asymmetric composition of supernova ejecta
(top) and the evolution of the observed brightness (as compared to
the brightness of the Sun) resulting from the model (bottom). The red
curve in the bottom panel shows the observation made from the direction
indicated by the red arrow in the top panel. For reference, the black
curve in the bottom panel shows the average over all directions.
|
|  |
Supernovae have driven the development of astronomy and fascinated
mankind for centuries as they are spectacular manifestations of the
limited lifetime of stars. Indeed, the Type Ia subclass of these
events, which is believed to originate from a thermonuclear explosion
of a star at the end of its evolution, is among the brightest events
that presently can be observed in the Universe. Such Type Ia
supernovae are as bright as billions of Suns. But what makes them
particularly valuable for astronomy is that not only are they bright
but their brightness seems to be very uniform. Therefore they have
been used as cosmic lighthouses to survey the Universe. This has led
to campaigns observing Type Ia supernovae in large numbers. Recently,
these observations showed that although the brightness of the majority
of events is rather uniform, some rare events exist which are more
than twice as bright as usual (e.g. Howell et al. 2006; Hicken et
al. 2007).
The explanation of these unusually bright Type Ia supernovae
challenges the theory of these objects. In the astrophysical standard
picture, a Type Ia supernova is due to a thermonuclear explosion of a
white dwarf star (the end stage of evolution of light and intermediate
mass stars) which accumulates matter from a normal companion star
until it reaches a mass 1.4 times that of the Sun. This so-called
Chandrasekhar mass marks the limit of its stability and the class of
models that assumes an explosion once this mass is reached is termed
"Chandrasekhar-mass models" of Type Ia supernovae. By fixing the
amount of material to fuel the thermonuclear explosion, this scenario
provides an elegant explanation of why Type Ia supernovae are so
uniform. This appealing theoretical picture is now challenged by the
new observations whose brightness may be taken as an indication that a
more massive object underwent a "Super-Chandrasekhar-Mass
explosion". In principle this may be possible if the white dwarf
rotates rapidly or if the exploding object is formed by two merging
white dwarfs. Such a variety of progenitors for Type Ia supernovae
potentially jeopardizes the cosmological application of these
events. But is it really inevitable? Recently, researchers at the MPA
proposed a scenario providing an explanation of the unusual brightness
within the conventional Chandrasekhar-mass model (Hillebrandt et
al. 2007).
The explosion is caused by a thermonuclear flame which ignites near the
center of the white dwarf star and propagates outwards transmuting its
material composed of carbon and oxygen to elements like
nickel, iron, and silicon. In particular, large amounts of radioactive
nickel are produced, the decay of which powers the supernova's
brightness. Conventional models
( Research Highlight October 2004)
assume that the thermonuclear flame ignites
evenly distributed around the center of the white dwarf. This leads to
a symmetric spread of the flame over the star and an isotropic
distribution of the radioactive nickel in the explosion ejecta. Such
models cannot account for the brightness observed in the peculiar
events. However, if the flame ignites off-center, its propagation is
asymmetric and so is the resulting distribution of the radioactive nickel
(e.g. Roepke et al. 2007). A large blob of this material may float to
the surface of the star and give rise to a very anisotropic
composition of the ejecta (the result of a three-dimensional
simulation of such an event is shown in Fig. 1).
Depending on the line of sight, such an explosion may substantially
vary in brightness. Seen along the direction of the nickel blob it
appears much brighter than seen from a perpendicular or reverse
direction. Does such a configuration lead to brightnesses sufficient
to explain the observations? This can be tested with a simple
representation of possible ejecta compositions. An example is shown in
the upper panel of Fig. 2. Here, the ejecta contains a blob of
radioactively unstable nickel (yellow) which is shifted upwards from
the center and is embedded in a shell of stable material (blue). What
would an astronomer observe from such an event? Typically, supernovae
brighten over a few days, reach peak brightness and then fade during
the following months. For the asymmetric model, the brightness of the
supernova was calculated as it would be observed from different
directions (lower panel of Fig. 2). As can be seen in the animation,
both the maximum and the evolution of the brightness depend on the
angle at which the supernova is seen (Sim et al. 2007). It is
brightest in the direction of the nickel blob and, in fact, as bright
as the recently observed extreme events. In this way, even these
brightest supernovae might be explained within the Chandrasekhar-Mass
model.
Stuart Sim, Friedrich Roepke, Wolfgang Hillebrandt, Daniel Sauer
Reference:
Hicken et al., 2007, ApJ, 669, L17
ADS
Hillebrandt W., Sim S. A., Roepke F. K., 2007, A&A, 465, L17
ADS
Howell et al., 2006, Nature, 443, 308
ADS
Roepke F. K., Woosley S. E., Hillebrandt W., 2007, ApJ, 660, 1344
ADS
Sim S. A., Sauer D. N., Roepke F. K., Hillebrandt W., 2007, MNRAS, 378, 2
ADS
|