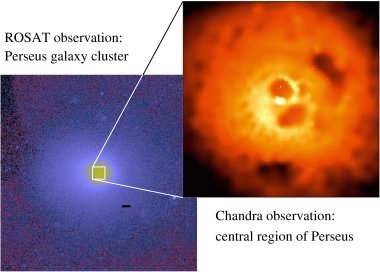 |
Figure 1: Cold core of the Perseus galaxy cluster. The blow-up (right)
shows the compressed gas. It is visible through its X-ray emission. (Left
exposure: ROSAT X-ray
satellite, right exposure: Chandra
X-ray satellite; image courtesy of NASA / IoA / A. Fabian et al.).
|
|
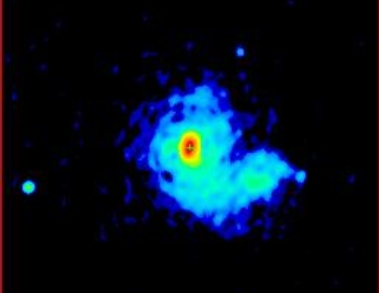 |
Figure 2: An extended radio source (a so-called radio mini-halo) in the
Perseus galaxy cluster which has a diameter of about 500,000 light years. This
image shows the color-coded radio emission at 1.4 GHz and was taken by the
American VLA telescope; image courtesy
of Pedlar et al. (1990).
|
|
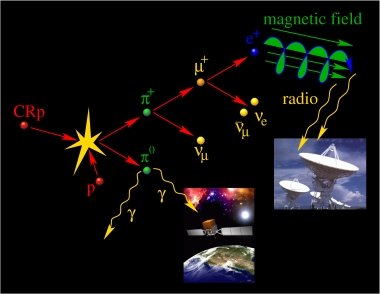 |
Figure 3: This diagram shows the high-energy particle reaction in the
proposed scenario. A proton traveling with approximately the speed of light
(cosmic ray proton, CRp) collides with a gas proton of the galaxy cluster and
produces an electrically charged pion (π+). This pion decays
successively into a muon (μ), into neutrinos (ν), and finally into an
electron or positron (e), dependent on the charge of the pion. This electron
(or positron) moves on a spiral orbit around the magnetic field lines in the
cluster of galaxies and generates radio emission which is visible on Earth with
radio telescopes like the VLA. A neutral
pion (π0) has the same probability as a charged one of being
generated. The neutral pion decays into two high-energy photons. This gamma-ray
emission can be detected with Cerenkov telescopes and should be detectable in
future satellite missions like GLAST. Images shown courtesy of GLAST / Spectrum Astro and NRAO / AUI /
NSF.
|
|
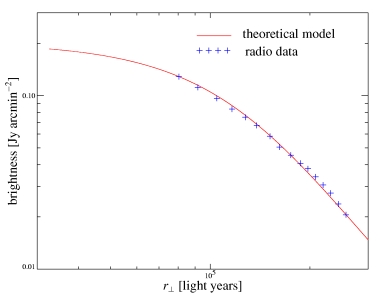 |
Figure 4: The radio brightness of the Perseus galaxy cluster as a
function of distance from the centre of the cluster (logarithmic
representation). The comparison of the observed radio data (blue crosses,
Pedlar et al. (1990)) and the new theoretical model shows a perfect
consistency.
|
|
|  |
Clusters of galaxies are the largest gravitationally bound objects in the
Universe. They consist of hundreds of galaxies and hot hydrogen gas which
emits X-ray radiation. They are created through violent mergers of groups and
smaller clusters of galaxies. During the phase of merging enormous amounts of
energy is released, which lead to a multitude of extended radio phenomena,
e.g. the formation of radio halos and radio relics (see Radio
Ghosts in Galaxy Cluster Collisions). In the following hundreds of millions
of years the galaxy clusters settle down again and the gas in the cluster
centers cools, collapses, and becomes compressed. This forms so-called cold
cores like that in the Perseus galaxy cluster (cf. Fig. 1). One whould expect
that in the settled clusters which have not received a new input of energy in
the last hundreds of million years no such extended radio emission to be
observable. However, radio telescopes detect diffuse emission in such objects
like the Perseus galaxy cluster (cf. Fig. 2). Where does the required energy
for the emission come from?
In the scenario proposed by Christoph Pfrommer and Torsten Enßlin cosmic ray
protons serve as an energy storage medium. These particles are able to store a
part of the energy released by group-cluster and cluster-cluster collisions in
the relatively thin gas inside clusters. This energy can be stored for
billions and billions of years and will not be emitted until the fast cosmic
ray protons collide with gas particles. The denser the gas within the centers
of clusters of galaxies the more frequent these particles collide. During such
collisions the fast protons create mostly pions. Many of the pions decay into
electrons and positrons which gyrate around magnetic field lines of the cluster
of galaxies. Because of the rapid circular motion the electrons radiate their
energy which can in turn be detected by radio telescopes after a long cosmic
journey (cf. Fig. 3).
Calculations show that comparatively few cosmic ray protons are necessesary to
reproduce the observed radio glowing. Moreover, there exists an astonishingly
good consistency between the predicted radio brightness distribution using this
model and the measured radio data (cf. Fig. 4).
The described particle interactions should also release high-energetic gamma
ray emission which can be detected on Earth using Cerenkov telescopes. The
recent gamma ray observation of the Virgo galaxy cluster using such a telescope
(HEGRA) could be a first
indication of the correctness of this scenario. Conclusive information about
this model is being expected with the launch of the future satellite mission GLAST (start date 2006).
Christoph Pfrommer & Torsten Enßlin
Further information:
|